The novel zeolite guest/host material, LiCl/H-STI (stilbite) assembly was synthesized by a thermal
dispersion method, and characterized in detail by powder XRD, 7Li MAS NMR, SEM/EDX, DTA and FT-IR. The loading threshold of LiCl was confirmed by XRD to be 0.14 g/g. The 7Li MAS NMR spectra were measured for a solution of LiCl, LiCl crystallites, LiCl/H-STI assembly, Li1 ion-exchanged STI(Li-STI) as well as LiCl dispersed on supports of zeolite ZSM-39, zeolite ZSM-5, silica gel and quartz.According to the chemical shifts, the states of Li1 can be identified as Li1 ions in solution, LiCl isolated in zeolite channels, Li1 cations balancing the framework charges and LiCl as a crystalline phase or cluster.Characterization results proved the LiCl guest was highly dispersed into the channels of the host at the threshold assembly loading.The interactions of LiCl with the zeolite framework and H2O are also discussed. The threshold loading LiCl/H-STI material was found to possess optimum humidity-sensitive performancewith a linear change of 4 orders of magnitude in electrical conductivity over the whole range of relativehumidity. In addition,it also shows satisfactory reversibility and fast responses to environmental moisture changes.
Introduction
Stilbite (type code STI, Na4Ca8[Al20Si52O144].56H2O), the first natural zeolite, was discovered in 1756 by Cronstedt.1 This zeolite possesses a two-dimensional interconnected channel system of 10-membered ring channels and 8-membered ring channels in its framework.2 The window size of the 10- membered ring channels along the [100] direction of the zeolite is 0.49 × 0.62 nm. The window size of the 8-membered ring channels (0.27 × 0.56 nm) along the [101] direction, which intersect with the 10-membered ring channels, is compatible with H2O molecules (0.26 nm). Therefore, the guest LiCl salt trapped in the 10-membered ring channels of the zeolite can absorb water through both channels.The applications of the natural STI zeolite (calcium form), discovered in north China, are limited due to its poor thermal stability. Usually, the framework of the natural zeolite collapses during dehydration at elevated temperature.3 For this reason, the zeolite has been poorly researched. In order to exploit the abundant natural resources of the zeolite in China, a series of investigations have been undertaken, with the structural characterizations and adsorption properties reported in detail.4–6 The thermal stability of the zeolite can be greatly enhanced from 250 。C to 700 。C, after exchanging the calcium ions of the parent Ca-STI zeolite by NH41 cations and then applying heat to produce both H-STI and NH3. The frame- work Si/Al ratio of H-STI (7.89) also increases compared to that of the parent (3.08) from the de-alumination of the framework after calcination.5 The host, H-STI zeolite, posse- sses 0.15 mL.g21 of water adsorption capacity at ambient temperature. Moreover, unusual adsorption selectivity for the length of carbon chain for various organic molecules has beenfound, which makes the zeolite an excellent candidate as a selective adsorbent.4
Humidity sensors have received a wide range of atten- tion due to their technological importance in a variety of applications; from air conditioning systems to medical and industrial equipment.7–11 The traditional materials used for sensor fabrications are solid electrolytes, polymers and porous ceramics. LiCl, a typical solid electrolyte, is one of the possible candidates for improving sensors.12–14 As a hydrophilic material, LiCl is extremely sensitive to changes in humidity. The concentration of LiCl solution corresponds directly to its conductivity. However, LiCl eventually becomes a solution upon exposure to high humidity, preventing its use in a solid state device. The electrical conductivity of LiCl solution is also affected by other factors, such as some contaminations. As a result, the quantitative relationship between humidity and conductivity is destroyed, which makes it impossible to apply the electrolyte practically, unless LiCl can be stabilized in the solid state.
This paper describes our attempts to use the H-form STI zeolite as the host to assemble and stabilize LiCl to synthesize a novel guest/host humidity-detecting material with a superior sensitivity to humidity.
Some inorganic compounds, such as oxides and salts, can spontaneously disperse into a support with high surface-to- volume capacity, such as Al2O3 and zeolite, via a thermal dispersion process (TD) reported by Xie et al.15 Researches have shown that these highly dispersed inorganic compounds are thermodynamically stable.16–18 Thus, this simple method may be utilized in the stabilization of LiCl.
In this paper, we report the preparation of the LiCl/H-STI guest/host material and the determination of the LiCl loading threshold, the maximum assembled amount of the guest in the host. The state of the LiCl guest in the host is characterized in detail and the relationship between synthesis procedure and humidity sensitivity of the material is also discussed.
Experimental Materials
The parent STI zeolite composed of Na0.2Mg0.1Ca8.4[Al17.2- Si54.8O144].65H2O, with a crystallite size of 5–10 μm, was collected in Beijing, China.4 No impurity phases were detected by X-ray diffraction, conducted at the laboratory of EXXON Inc. in New Jersey, USA. The parent zeolite was treated three times at 95 。C, stirred for 2 h in a solution of 1 M NH4Cl at a zeolite/solvent ratio of 1/10, washed with de-ionized water, and then collected by filtration. After the final ion exchange treatment to obtain the NH4-STI, the material was washed with de-ionized water until no free Cl2 ions were detected in the filtered water and dried at 80 。C for several hours. The NH4- STI was heated from room temperature to 550 。C at a rate of 10 。C min21 in N2 and calcined at 550 。C for 2 h in an oven with a programmable temperature controller, then allowed to cool, producing the H-STI zeolite. The Langmuir surface area and the microporous volume were determined with the adsorption/desorption of N2 at 77 K using a Micromeritics ASAP 2000 instrument and were found to be 425 m2 g21 and 0.20 cm3 g21, respectively.4
H-STI was mixed with measured amounts of LiCl.H2O (analytical grade, Shanghai Chemical Reagent Co.) and de- ionized water, and then stirred at room temperature for 20 minutes to form a turbid mixture. The mixture was dried at 100 。C to get sample XX0 (the subscript 0 here refers to the samples without calcination). After calcination of sample XX0 at 250 。C for 16h, sample XXTD, (subscript TD refers to thermal dispersion method), was obtained. The samples with LiCl loadings of 0.00 g/g, 0.08 g/g, 0.11 g/g, 0.14 g/g, 0.17 g/g, 0.20 g/g and 0.45 g/gare expressed as samples A,B,C,D,E, F, and G, respectively. If not specified, the subscripts TD and 16 are omitted hereafter. For example, sample D represents the sample prepared by the TD method with 16 h of calcination at 250 。C.
To investigate both the structures and the properties of the materials above, another series of samples prepared by a mechanical grinding method (MG) were made in parallel for comparison. In these samples, H-STI zeolite combined with a certain amount of LiCl.H2O was ground in anagate mortar for 30 minutes to obtain the sample XXMG.
H-STI zeolite and a solution of 0.2 M LiCl were mixed with a solid/liquid ratio of 1/20. After undergoing four ion-exchange treatments at 95 。C, a Li ion-exchanged STI zeolite (Li-STI) sample was obtained. In order to investigate the state of the assembled LiCl, other LiCl/Host systems, such as LiCl/ZSM- 39, LiCl/ZSM-5, LiCl/Silica gel and LiCl/Quartz, were pre- pared by the same TD method as with LiCl/H-STI, except for LiCl/ZSM-5 which was calcined at 400 。C.
Characterization
X-Ray powder diffraction (XRD) patterns of the samples were collected using a Rigaku D-MAX/II A automatic X-ray diffractometer with Cu Ka radiation. Slow scanning XRD was conducted in the range of 27–36。(2θ) at the rate of 1。(2θ) min21. The morphology and the size of the assembled zeolite crystallites were investigated with a PHILIPS XL30 scanning electron microscope (SEM) at 20 KV. Chlorine elemental analysis was performed on the EDX energy diffraction spectrometer of the SEM. FT-IR spectra were obtained with a Nicolet 5SXC FTIR spectrometer at room temperature. The KBr disk method was employed (sample/KBr = 1/100) with a scan range of 400–1800 cm21, and a precision of 4 cm21. The DTA curves were measured from room temperature to 900 。C at a rate of 10 。C min21 in N2 with a Rigaku PTC/10A thermal analyzer. About 10 mg of the samples were used for each test. Magic angle spinning nuclei magnetic resonance (MAS NMR) spectra of the samples were measured on a Bruker MSL
300 spectrometer operated at 116.46 MHz for 7Li. All MAS NMR spectra were recorded with a 4 mm double-bearing MAS probe at spinning frequencies of 10 kHz, corresponding to a π/2 pulse width of 2.5 μs. The spectral width was 20 ppm. The radio frequency field was 2.33 kHz. The recycle time was 3 s. For each measurement, 2000 spectra were accumulated. To ensure the dehydrated samples investigated did not come in contact with water, they were sealed in a tube in an absolutely dry glove box under nitrogen atmosphere. 1.0 M LiCl aqueous solution and dehydrated LiCl crystallites were used as a liquid-state reference and a solid-state reference, respectively.
Electrical conductivities
All powder samples used for resistance measurements were pressed into uniform cylinders of Ø 20 × 1.4mm, and then placed in a sealed humidistat for more than 12 hunder ambient temperature to ensure equilibrium with background moisture. An EG&G AC-impedance instrument consisting of a M273A potentiostat and a M5210 lock-in amplifier was connected to two copper electrodes taped to the cylindrical sample with a pair of wires going through the sealed humidistat. The AC frequency used was varied from 0.05 to 100 KHz. The resistance data were collected with the M398 impedance software.
To test the in situ humidity response time, sample tablets described above were first placed in the humidistat at RH (relative humidity) = A for half an hour, then quickly shifted to another humidistat at RH = B. The attached CHI660 workstation recorded the response signals from the samples and showed humidity changes relative to the changes in conductivity.
Results and discussion XRD patterns
XRD patterns for the TD series samples are given in Fig. 1(I). The XRD patterns of samples B,C, and D are similar to that of
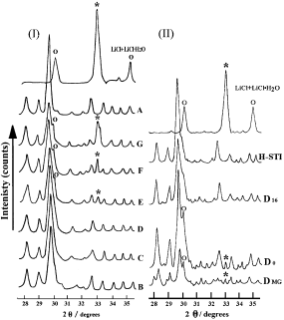
Fig. 1 (I) XRD patterns of LiCl 1 LiCl.H2O and the samples for thermal dispersion (TD) series with various loadings of LiCl: A, H-STI; B, 0.08 g/g; C, 0.11 g/g; D, 0.14 g/g; E, 0.17 g/g; F, 0.20 g/g; G, 0.45 g/g. (II) XRD patterns of LiCl 1 LiCl.H2O, H-STI, D16 (calcination at 250 。C for 16 h), D0 (without calcination), and DMG (with 0.14 g/g of LiCl loading prepared by mechanical grinding methods). (o — LiCl; * — LiCl.H2O.)
A (host, H-STI zeolite). The characteristic peaks are consistent with the data reported in references.4,5 This result implies the guests in samples B, C or D were already highly dispersed within the zeolite host. As the loading of LiCl was gradually increased, the characteristic peaks assigned to crystalline LiCl and LiCl.H2O appeared in the XRD patterns of samples E, F and G, with the relative intensity of these peaks increasing in each sample. This indicates the agglomeration of the residual LiCl (or LiCl.H2O) is present in a crystalline phase outside the host as opposed to dispersing within the zeolite.15,16,19 The precise state of the guest deduced above was further investigated with other techniques including SEM/EDX, MAS NMR, FT-IR and DTA as described in the following parts. According to the XRD patterns of the TD sample series, the dispersion threshold of LiCl loaded in H-STI approximates to 0.14 g/g. Therefore, sample D is described as the threshold sample.
The assembly process is probably influenced by various factors, such as the preparation method, the dispersion time and the reaction temperature. Thus, several samples with the threshold loading (0.14 g/g ofLiCl) were prepared by different methods for further investigation. The XRD patterns of these samples are shown in Fig. 1(II). In the XRD pattern of sample DMG, prepared by a mechanical grind (see Fig. 1(II)-DMG), a diffraction peak arising from LiCl and a small peak assigned to LiCl.H2O appeared at 30.09。/2θ and 33.00。/2θ, respectively, while the relative intensities of the diffraction peaks of the host were simultaneously weaker. The results show that this guest exists in the crystalline phase mixed with the host crystallites instead of dispersing into the zeolite channels, which partially destroyed the zeolite structure and decreased the crystallinity of the host. In the XRD pattern of sample D0 (see Fig. 1(II)-D0), which was prepared by stirring smoothly the host–guest mixture without calcination, the diffraction peaks of crystalline LiCl and LiCl.H2O show a certain amount of the salt crystals still exist. The intensity of the diffraction peaks for the host remains the same in this sample. This indicates the stirring method introduces more LiCl into the zeolite structure and maintains the crystallinity of the zeolite compared to a mechanical grind method. The characteristic diffraction peaks of the guest disappear completely in the XRD pattern of sample D16, which was obtained by calcination of sample D0 at 250 。C for 16 h (see Fig. 1(II)-D16). No visible changes of the diffraction angles or the intensity for the host were observed after the calcinations. This suggests the high dispersion of the guest in the host. The comparison above confirms thermal dispersion results in high quality samples.
The XRD patterns (not shown here) of other LiCl/Host systems prepared under the same conditions as LiCl/H-STI provide additional evidence for dispersive states of the LiCl guest.
The XRD pattern of LiCl/ZSM-39 (not shown here) exhibits no peaks belonging to LiCl, indicating the guest has dispersed onto the ZSM-39 support. ZSM-39 is a member of the Clathrasils. In a unit cell of the Clathrasil zeolite, the Si–O framework is constructed with eight [51266] and eight [512] cages. The [51266] cage is composed of a 5-membered ring and a 6-membered ring window, which is too small to let the LiCl enter. This suggests the dispersed LiCl exists outside the framework of the host. We call this kind of LiCl ‘‘outside framework-clusters’’ .
The fact that no peaks assignable to LiCl are found in the XRD pattern of LiCl/Silica gel (not shown here) is possibly due to the huge surface area of the amorphous host. The micro- crystals of LiCl that could successfully disperse into the channels and the cages with various sizes are defined as ‘‘inside framework-crystallites’’ .
With the quartz sample as a very dense substrate, LiCl crystallites were only able to disperse on the surface of the host.This was proven by sharp peaks assigned to LiCl crystals observed in the XRD pattern of the system (not shown here).
ZSM-5 zeolite possesses intersecting channels and sinusoidal channels along the [100] direction with a window size of 0.53 × 0.56 nm and straight channels along the [010] direction with a window size of 0.53 × 0.56 nm. The guest may have dispersed into both the channels and the cages formed in the intersection areas. We call this kind of LiCl ‘‘inside channel/ cage-clusters’’ . The peaks assigned to LiCl are shown in the XRD pattern of the sample prepared by calcination at 250 。C (not shown here). With increased temperatures to 400 。C, these peaks disappear completely. The dispersion at higher tempera- ture implies the LiCl guest is more easily assembled into H-STI than ZSM-5.
Theoretical calculation of LiCl amount
To exclude the possibility of the loading LiCl guest employed as the Li1 ion-exchanged source, we utilized the computer simulation method to determine the required amount of LiCl on 100% Li1 ion-exchanged reaction. From the 29Si NMR spectra (not shown here),5 we found the framework Si/Al ratio of the H-STI is 7.89. Based on the assumption that 100% ion- exchanged reaction means all the negative charges from the framework are balanced by Li1 ions, we can obtain the LiCl amount required for providing Li1 ions in the ion-exchanged reaction. With the expression given below (Equation 1), it is 0.078 g LiCl per gram of H-STI (g/g), much less than the threshold of the assembly LiCl/H-STI, which is 0.14 g/g.
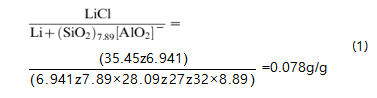
7Li MAS NMR
7Li MAS NMR spectra depicted in Fig. 2(1) shows only one signal for the LiCl/H-STI (sampleD) with the absence of visible spinning side bands. It implies that all of the assembled LiCl
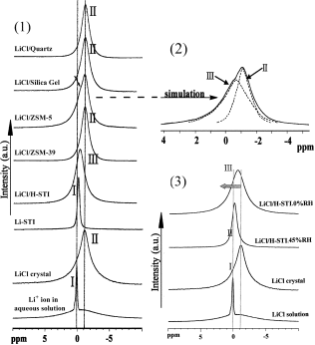
Fig. 2 (1) 7Li MAS NMR spectra of Li containing samples; (2) simulation of 7Li MAS NMR spectra of LiCl/ZSM-5; (3) 7Li MAS NMR spectra of LiCl/H-STI at different humidities.
Table 1 Chemical shifts of 7Li MAS NMR on various samples
Sample | RH (%) | δ(ppm) | Δδ|x-sol.|a (ppm) | Δδ|x-cry.|b (ppm) | FWHM/ Hz | |
LiCl (Sol.)LiCl (Cry.)Li-STIcLiCl/H-STId LiCl/H-STId LiCl/ZSM-39 LiCl/ZSM-5eLiCl/Silica gel LiCl/Quartz | 0000450000 | 0.00 21.09 20.078 20.37 20.12 21.12 21.18 20.65 21.12 21.12 | —1.18 0.17 0.46 0.21 1.21 1.27 0.74 1.21 1.21 | 1.18—1.01 0.72 0.97 0.03 0.09 0.44 0.03 0.03 | 202505018811415975137180158 | |
a Δδ|x-sol.| – the difference between Δδx, the chemical shift of 7Li in a given sample, and Δδsol, the chemical shift of 7Li in an aqueous solu- tion of LiCl. b Δδ|x-cry.| – the difference between Δδx, the chemical shift of 7Li in a given sample, and Δδcry, the chemical shift of 7Li in LiCl crystals. c Li1 ion-exchanged zeolite. d LiCl/H-STI sample with threshold loading. e There are two peaks in the 7Li NMR spectrum of LiCl/ZSM-5. |
within the zeolite channels are in a similar chemical environ- ment. In addition, the signal exhibits a small but detectable high-field shift compared to that of an aqueous solution of LiCl and a low-field shift compared to that of the crystalline LiCl (see Table 1). The signal appearing at higher field compares to that of Li-STI, with a broader line. This indicates that Li cations in LiCl/H-STI are located in a different chemical coordination environment compared to the other three Li- containing species. Variations in both chemical shift and the line width of the 7Li signal are also notable in the threshold sample under different levels of ambient humidity (see Fig. 2(3)). Under 0% RH, Li cations reveal a high-field chemical shift with a broader line (FWHM = 188 Hz), somewhat similar to that in the spectrum of the LiCl crystal. Increasing the humidity to 45%, the signal becomes narrower (FWHM = 114 Hz) and shifts to a lower field, similar to that of the Li cations in an aqueous solution. This dynamic change agrees with the humidity-sensing process of the LiCl assembled in the host zeolite. One possible explanation is that the electron clouds of Cl2 anions have a screening effect on the Li cations, leading to a relatively high field signal of the LiCl crystal. When LiCl is dispersed into the channels of the zeolite, the electrostatic force is partially weakened because of the inter- action of the cations with the O22 anions in the framework. As a consequence, the resonance of 7Li shifts to a lower field with the lowered shielding. After adsorbing moisture, Li1 cations in the channels are surrounded by water molecules. As a result, the interaction between Li1 and Cl2 is weakened to give a downfield shift. The narrower line shape is due to the mobility of the Li cations. The NMR results provide evidence of the humidity sensitivity of the assembly material.
7Li MAS NMR spectra for all LiCl/Host systems are shown in Fig. 2(1) and listed in Table 1. It can be clearly seen that 7Li signals of all investigated samples are in the chemical shift range of 21.18 ppm < δ < 0.00 ppm. The 7Li signal of an aqueous solution of LiCl is at 0.00 ppm (State I). The signals forLiCl crystals, LiCl/ZSM-39,LiCl/Silica gel and LiCl/Quartz are at δ = 21.10 ppm. It can be concluded that Li cations in these samples exist as either crystallites or clusters of LiCl (State II), consistent with the results of XRD. The 7Li signals are in the range of 20.5 ppm < δ < 0.0 ppm for Li-STI and LiCl/H-STI, suggesting the cations are isolated from each other (State III) in the zeolite channels. This isolated state would be either Li1 ions in Li-STI existing at the zeolite cations position to balance the negative charge of the framework or Li1 in the assembly acting as the counterpart of Cl2 in the highly dispersed state of LiCl in the zeolite channels of LiCl/H-STI. While in LiCl/H-STI, the ions act as the counterpart of Cl2 inthe highly dispersive state of LiCl in the zeolite channels. The signal of LiCl/ZSM-5 possesses a small shoulder, which can be simulated to two peaks at 21.18 and 20.65 ppm, respectively (see Fig. 2(2)). Li cations of LiCl/ZSM-5 can be interpreted to two states, state II (21.18 ppm, crystalline or cluster state) and state III (20.65 ppm, highly disperse state). The former suggests the LiCl is present as clusters in the larger cavities at the intersections of the sinusoidal channels and straight channels. The latter indicates guest LiCl is in isolated forms highly dispersed in the channels, which is consistent with the results from XRD. As a powerful tool for determining the local environment of Li ions on various supports, the NMR tech- nique reveals the highly dispersive nature of LiCl in LiCl/H-STI with threshold loading.
SEM images and EDX spectra
SEM images (not shown here) of typical samples show that the crystallite morphology of sample B is very close to that in sample A, with only plate-like crystallites of STI zeolite visible. The cubic crystals of LiCl and the tetragonal crystals of LiCl.H2O can be observed in the image of sample G. This suggests the guest is highly dispersed into the channels of the host zeolite when the loading is less than the threshold.20 When the loading exceeds the threshold, the residual LiCl crystallites form outside the zeolite channels.
As shown in Fig. 3, the EDX spectra of the threshold loading LiCl/H-STI exhibited a considerable amount of chlorine element. No chlorine signal was observed in the spectrum of Li-STI. This result gives further evidence that LiCl/H-STI assembly is different from Li-STI. It also agrees with the results of 7Li MAS NMR.
FT-IR
Fig. 4 presents the FT-IR spectra of the host, the guest, the TD series samples, and the MG series samples. In the spectrum of the host (see Fig. 4(A)), the adsorption band at 1636 cm21 is assigned to the vibration of the lattice water and hydroxyl. The vibrations of external linkages, such as the asymmetrical stretch at ~ 1155 cm21, the symmetrical stretch at ~ 777 cm21, and the double ring vibration at ~ 588 cm21 are sensitive to the changes of the framework structure. The main FT-IR vibra- tions such as the asymmetrical stretch at ~ 1065 cm21, the symmetrical stretch at ~ 716 cm21, and the T–O band at
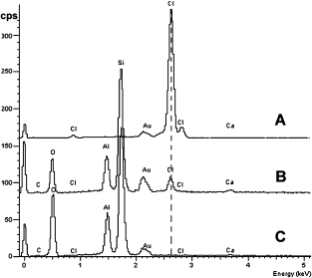
chlorine element content. A. Pure LiCl crystal; B. LiCl/H-STI zeolite assembly; C. ion-exchanged Li-STI zeolite.
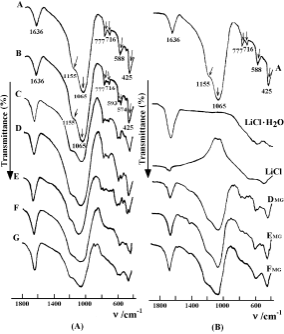
~425 cm21, corresponding to the vibration of the internal tetrahedron, are insensitive to these changes.21 A strong peak characteristic of the lattice water at ~ 1636 cm21 appears in the IR spectrum of LiCl.H2O (see Fig. 4(B)), but is absent in the spectrum of LiCl.
In the spectra of the TD series samples (see Fig. 4(A)), increasing the loading of LiCl on the zeolite results in an obvious enhancement of the intensity of the vibration at 1636 cm21. In the spectra of samples B, C, or D, in which the loading of LiCl is less than the threshold, the wave number and the sharpness of the vibrations are almost unchanged in comparison with those of the host, indicating the framework of H-STI has remained intact. Close inspection of the spectra reveals the single peak assigned to the double ring mode at ~ 588 cm21 splits into two peaks at ~ 593 cm21 and ~ 574 cm21, respectively. This split becomes more pronounced as the loading increases. This could be related to the interaction between the framework and the LiCl entering the channels of the zeolite. In the case of samples E, F or G, in which LiCl loading exceeds the threshold, the vibrations of the zeolite framework are obviously weakened and have become broader. Moreover, the characteristic vibrations of LiCl and LiCl.H2O are present and have become more intense in the range of 400– 900 cm21. The vibrations at 777 cm21 and 588 cm21 for the host gradually disappear (see Fig. 4(A)-E, F and G).12 These results indicate the residual crystallites of LiCl accumulating on the surface of H-STI crystallites may give rise to a decrease in zeolite crystallinity. The profiles of the spectra for the samples E, F and G are very similar to those of the spectra for the MG series samples (see Fig. 4(B)). This further proves some samples of the TD series, in which the loading of LiCl exceeds the threshold, are likely a mixture of the crystallites of the assembly guest/host material and the crystallites of the LiCl guest.
DTA
Fig. 5 gives the DTA curves for the samples. The DTA curve of LiCl.H2O has endothermic peaks in two regions: strong and sharp peaks assigned to the dehydration of LiCl.H2O in the
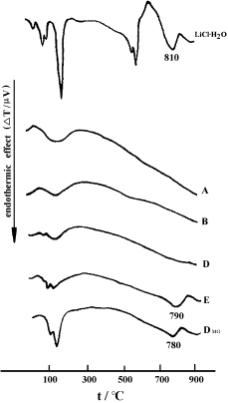
temperature range of 100–300 。C, and a peak of medium intensity attributed to the melting point of LiCl at 810 。C. The DTA curve for H-STI shows only one broad and weak peak in the temperature range of 100–300 。C, due to dehydration.4,22,23 In the curves of the TD series samples in which the loading is less than the threshold, such as samples B and D, only one dehydration peak is present in the temperature range of 100– 300 。C. It is similar to that of the host (sample A). This suggests the loaded LiCl (or LiCl.H2O) is highly dispersed into the zeolite channels without free crystallites existing outside the channels. Endothermic peaks at 790 。C and 780 。C are present in the DTA curves of sample DMG and sample E respectively, both of which have a higher LiCl loading than the threshold. The temperatures of these peaks are close to 810 。C,the melting point of LiCl, which suggests the presence of the free crystallites of the salt.17 The dehydration peak in the curve of sample DMG is also much sharper than that of sample E. It reveals that even in the sample prepared by TD with high guest loading, the amount of free LiCl crystallites is still obviously less than those in the sample prepared by MG with low guest loading. The results once again confirm that most of the guest prefers to disperse into the zeolite channels of H-STI under thermal dispersion conditions.
Humidity-sensitivities
The humidity–resistance characteristics of some typical samples are given in Fig. 6. It seems the conductivity, which is inversely proportional to the resistance of the humidity sensitive material, was affected by the assembly’s composition. Under anhydrous conditions, the measured conductivity of the pure host is less than 10212 S.cm21. With increased guest loading, the conductivity of the samples grows to a maximum of 3.7 × 1028 S.cm21 on the threshold sample. This is 4 orders of magnitude higher than that on the guest. As the loading exceeds the threshold, the conductivity declines. Excessive
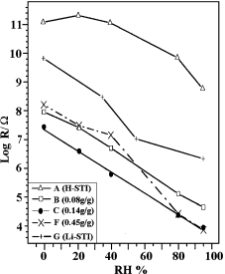
loading of LiCl in the host results in the agglomeration of the residual LiCl crystal, which could hinder the electric current continuity of the guest/host material. After exposure to a moist atmosphere, the assembly material begins to adsorb the mois- ture in air. Although slightly hydrophilic, the host and the ion- exchanged Li-STI zeolite are restricted in their application by the high bulk resistance and the poor linearity of their humidity sensitivity. The sample with the threshold loading possesses the best humidity sensitivity with log R/Ω = 3.9–7.4 in the humidity range of 0–95% RH, and the best linearity when compared to the higher impedance sample with 0.08 g/gofLiCl loading and the poorer linearity sample with 0.45 g/g of LiCl.
The reversibility of humidity changes on LiCl/H-STI is shown in Fig. 7A. Here the relative humidity was elevated from 0% RH to 95% RH by about a 20% increment in each step and then dropped back to 0% RH at the same rate. The total conductivity change at 0% RH is less than 0.3 orders of magnitude, showing a satisfactory reversibility for desorption on the threshold sample.
The time response characteristics of the threshold sample were examined under rapid humidity changes from 0% to 80% RH (adsorption process) and then back to 0% (desorption process), indicating the material exhibits a quick response to humidity changes. The response times for adsorption and desorption are within 26.5 s and 38.6 s, respectively, in the testing RH range of 63.2% change (see Fig. 7B).
The excellent humidity-sensing qualities of LiCl/H-STI zeolite assembly confirm its potential as a humidity detecting material.24,25 Work in the field of assembly zeolite sensing materials continues in this lab.
Conclusion
A novel assembly Guest/Host material, LiCl/H-STI, was successfully prepared by a thermal dispersion method based on a natural two-channeled zeolite, stilbite. Characterization by XRD, 7Li MAS NMR, SEM/EDX, FT-IR and DTA reveal the guest (LiCl) is highly dispersed into the zeolite channels of the host (H-STI), with the threshold LiCl loading of 0.14 g/g. The excellent sensitivity, linearity and fast response to humidity changes show the material is promising for practical applica- tion. The 7Li MAS NMR spectra provide detailed information about the dispersion states of LiCl on various supports as well.
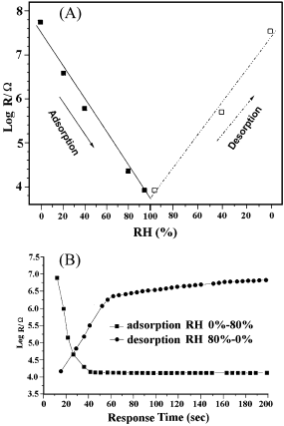
Supporting information available
There are five figures with the following captions:
A-F-1. XRD patterns of ZSM-39 system: (a). ZSM-39; (b). 0.14g/g LiCl/ZSM-39 (250 。C, 16h).
A-F-2. XRD patterns of silica gel system: (a). Silica gel; (b). 0.14g/g LiCl/silica gel (250 。C).
A-F-3. XRD patterns of quartz system: (a). 0.14g/g LiCl/ quartz (250 。C 16h); (b).quartz.
A-F-4. XRD patterns of ZSM-5 system: (a). ZSM-5; (b). 0.14g/g LiCl/ZSM-5 (400 。C 16h); (c). 0.14g/g LiCl/ZSM-5 (250 。C 16h).
A-F-5. SEM images of: a- sample A (H-STI); b- sample D (0.14g/g); c- sample G (0.45g/g).
Acknowledgements
This work was supported by Project 20073010 of National Natural Science Foundation of China, and The Nano- Materials Foundation of Shanghai (Projects 0213NM042). We also gratefully acknowledge Dr. Richard K. Baldwin for his participation in this paper.
References
1 A. Alberti and C. Vezzalini, in Natural Zeolites, ed. L. B. Sand and F. A. Mumpton, Pergamon Press, New York, 1978, pp. 85–98.
2 Ch. Baerlocher, W. M. Meier and D. H. Olson, Atlas of Zeolite Structure Types, Fifth Revised Edition, Elsevier, Guildford, 2001, p. 258.
3 E. Passaglia, Mineralogische Tschermaks und Petrographische Mitteilungen, 1980, 27, 67.
4 J. Li, J. Qiu, Y. J. Sun and Y. C. Long, Microporous Mesoporous Mater., 2000, 37, 365.
5 J. Qiu, G. Q. Zhang, Y. J. Sun, H. W. Jiang and Y. C. Long, Acta Chim. Sin. (Chin. Ed.), 1999, 57(4), 377.
6 J. Li, J. Qiu and Y. C. Long, Acta Chim. Sin. (Chin. Ed.), 2000, 58(8), 988.
7 T. Munoz Jr. and K. J. Balkus Jr., Chem. Mater., 1998, 10, 4114.
8 P. Shuk and M. Greenblatt, Solid State Ionics, 1998, 113–115, 229.
9 M. Pelino, C. Cantalini, H. T. Sun and M. Faccio, Sens. Actuators B, 1998, 46, 186.
10 W. M. Qu, W. Wlodarski and J. U. Meyer, Sens. Actuators B, 2000, 64, 76.
11 C. N. Xu, K. Miyazaki and T. Watanabe, Sens. Actuators B, 1998, 46, 87.
12 X. T. Li, C. L. Shao, S. L. Qiu, F. S. Xiao and H. Ding, Chem. J. Chin. Univ., 2000, 21(8), 1160.
13 L. B. Kong, L. Y. Zhang and X. Yao, J. Chin. Ceram. Soc., 1998, 26(4), 483.
14 M. K. Jain, M. C. Bhatnagar and G. L. Sharma, Sens. Actuators B, 1999, 55, 180.
15 Y. C. Xie and Y. Q. Tang, Adv. Catal., 1990, 37, 1
16 C. B. Wang, Y. C. Xie and Y. Q. Tang, in Proceedings of the 12th International Zeolite Conference, Baltimore, Maryland, eds. M. M. J. Treacy, B. K. Marcus, M. E. Bisher, J. B. Higgins, Material Research Society, Pennsylvania, U.S.A., 1998, p. 175.
17 F. S. Xiao, S. Zheng, J. M. Sun, R. B. Yu, S. L. Qiu and R. R. Xu, J. Catal., 1998, 176, 474.
18 Y. C. Xie, N. F. Yang, Y. J. Liu and Y. Q. Tang, Sci. Sin. Ser. B, 1982, 8, 673.
19 Y. J. Liu, Y. C. Xie, J. Ming, J. Liu and Y. Q. Tang, J. Catal. (Chinese), 1982, 3(4), 262.
20 Y. F. Zhang, Y. C. Xie, Y. Zhang, D. L. Zhang and Y. Q. Tang,
Sci. Sin. Ser. B, 1986, 8, 805.
21 H. G. Karge, Microporous Mesoporous Mater., 1998, 22, 547.
22 P. M. Rao, Adv. Catal.: Proc. Natl. Symp. Catal. 7th, 1985, 155.
23 A. Dyer and H. Faghihian, Microporous Mesoporous Mater., 1998, 21, 39.
24 J. Zou, H. Y. He, J. P. Dong and Y. C. Long, Chem. Lett., 2001, 810.
25 J. Zou and Y. C. Long, ZL 01112692.2, 2001.